Northwestern University Physicists Build Bridges for Quantum Communication
In a recent study, physicists from Northwestern University have introduced a novel approach to sustain communication within a dynamic and unpredictable quantum network. By reconstructing the vanishing links of entangled photons, the researchers discovered that the network ultimately stabilizes into a consistent—albeit altered—state.
Understanding Quantum Networks and Their Challenges
Quantum networks are more than just an intriguing idea; they signify a groundbreaking advancement in our understanding of communication and computation. Fundamentally, these networks utilize the principles of quantum mechanics to create secure communication channels, taking advantage of the phenomenon known as quantum entanglement. This aspect is particularly captivating! Consider two particles that are so intricately linked that the condition of one immediately reveals the condition of the other, regardless of the distance separating them. It resembles a form of cosmic telepathy between particles, paving the way for extremely secure communications that traditional systems can only aspire to achieve.
The path to unlocking the full capabilities of quantum networks is fraught with challenges. A major obstacle is the delicate nature of entangled photons. These particles are similar to fleeting shooting stars—striking and promising, yet they disappear after a single use. Consequently, each time a photon is utilized for communication, it is lost, resulting in interruptions within the network. This can be compared to crossing a bridge and setting it ablaze behind you; once that connection is utilized, it is no longer available for subsequent use. This issue not only disrupts communication but also complicates the network’s ability to maintain stability in ever-changing environments where users are continuously engaging with one another.
In addition, keeping connections stable is vital in a fragile quantum network. As more users join, the network risks breaking apart. Losing too many connections can make it stop working, while having too many can overload it. Researchers compare it to balancing on a tightrope, where finding the right balance is crucial for the network to work efficiently and reliably.
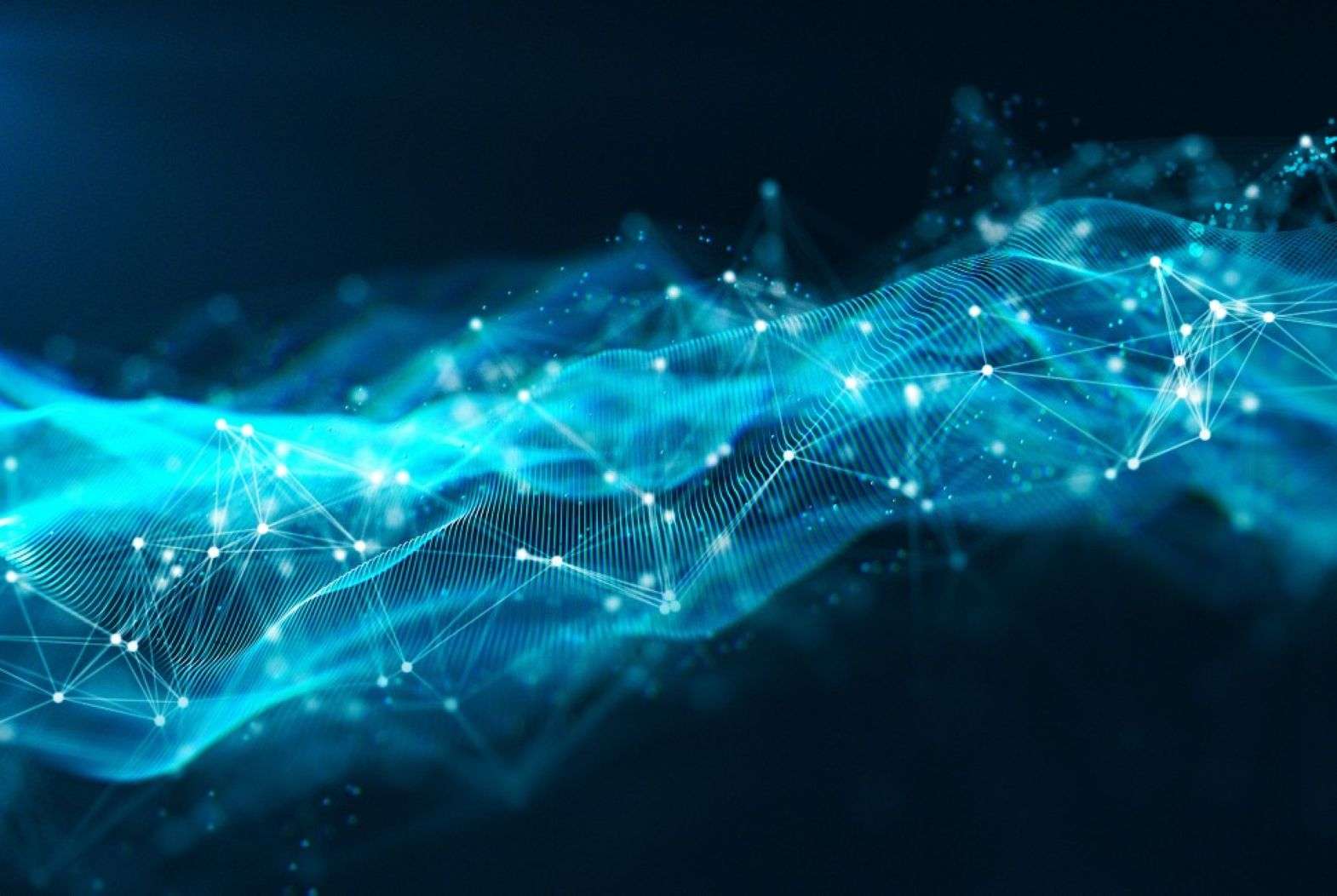
Innovative Strategy by Northwestern University
To tackle these issues, a team of researchers at Northwestern University, led by physicist István Kovács, has created a model aimed at effectively managing network connections. Their findings indicate that the crucial factor is incorporating an adequate number of connections to maintain network functionality. However, increasing the number of connections excessively can lead to significant costs and strain on resources. Conversely, having too few connections leads to a disjointed network that fails to meet user demand.
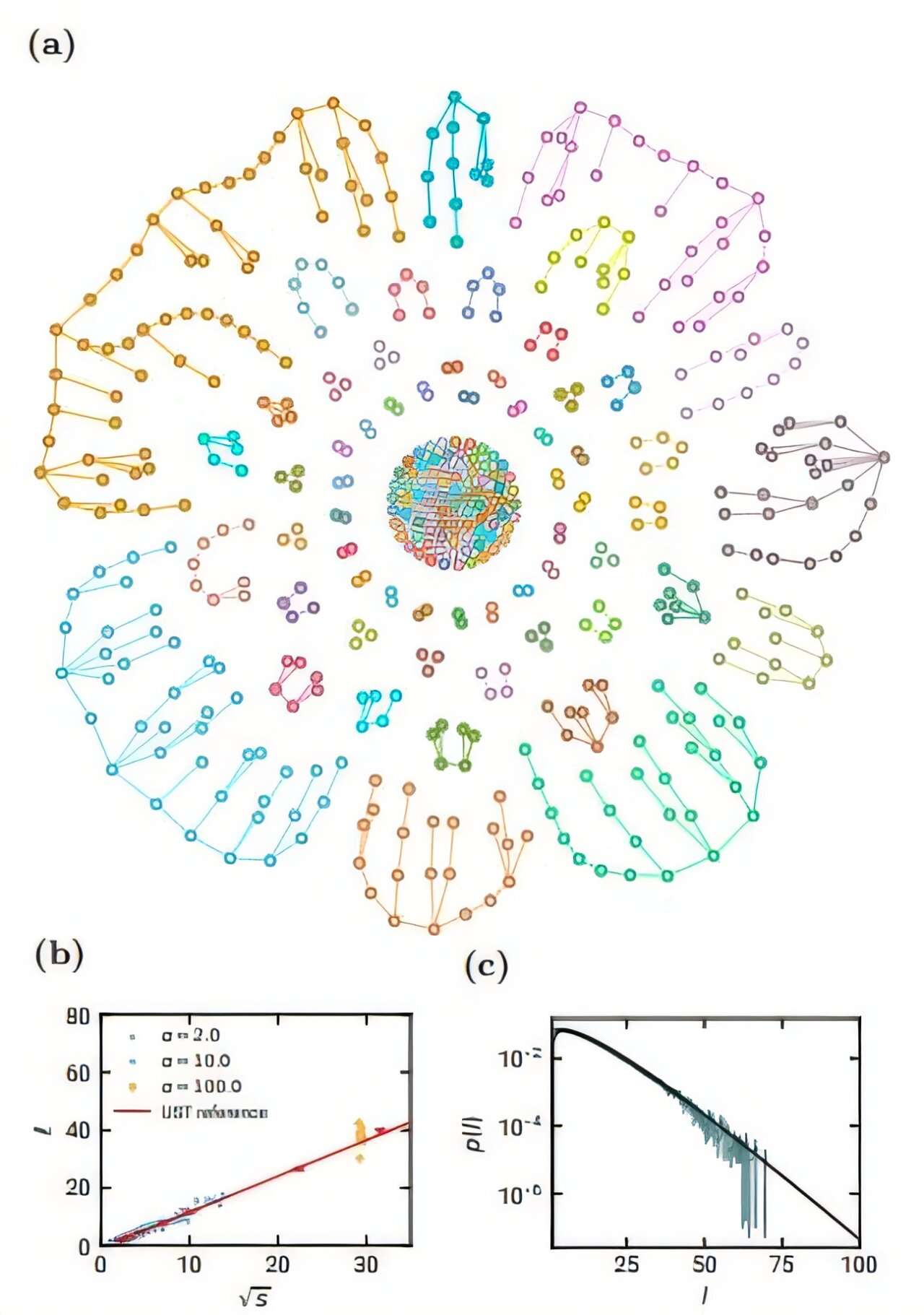
These discoveries could pave the way for creating ideal quantum networks, enabling super-fast computing and highly secure communication. The research is published in journal Physical Review Letters.
Northwestern’s István Kovács, the study’s senior author, said, “Many researchers are putting significant efforts into building larger and better quantum communication networks around the globe.”
“But, as soon as a quantum network is opened up to users, it burns down. It’s like crossing a bridge and then burning it down behind you. Without intervention, the network quickly dismantles. To tackle this problem, we developed a simple model of users. After each communication event, we added a fixed number of bridges, or links, between disconnected nodes. By adding a large enough number of links after each communication event, we maintained network connectivity.”
Kovács, a specialist in complex systems, serves as an assistant professor of physics and astronomy at Northwestern University’s Weinberg College of Arts and Sciences.
Quantum Network of disappearing links
Quantum networks operate by utilizing quantum entanglement, a phenomenon where two particles remain interconnected regardless of the physical distance between them. Xiangi Meng, an expert in quantum communication and one of the lead authors of the study, characterizes entanglement as a “spooky” yet highly effective resource. During the study, Meng was a research associate in the Kovács group but has since taken on the role of assistant professor of physics at Rensselaer Polytechnic Institute in New York.
“Quantum entanglement is the spooky, space-time-defying correlation between quantum particles,” Meng said. “It’s a resource that allows quantum particles to talk to each other, so they can perform complex tasks together while ensuring no eavesdropper can intercept their messages.”
When two computers connect through entangled links, those links dissolve after use. The communication process itself changes the link’s quantum state, rendering it useless for subsequent communications.
“In classical communications, the infrastructure has enough capacity to handle many, many messages,” Kovács said. “In a quantum network, each link can only send a single piece of information. Then it falls apart.”
Finding the Perfect Balance
Kovács and his team developed a simplified model of user behavior within a quantum network to gain deeper insights into how networks operate under continuous change. Initially, they allowed users to randomly choose other users for communication. Subsequently, they identified the shortest and most efficient communication route between the selected users and removed all the connections along that route. This process resulted in “path percolation,” where the network progressively deteriorated with each communication interaction.
Kovács and his team investigated this issue and proposed a solution. Through their modeling, they determined the precise number of links that must be added following each communication event. This number lies at the critical threshold between preserving the network’s integrity and causing it to break apart. Interestingly, they discovered that the crucial value corresponds to the square root of the total number of users. For instance, if the network comprises 1 million users, 1,000 links need to be reintroduced for every single qubit of information transmitted across the network.
“It would be natural to expect that this number increases linearly with the number of users, or maybe even quadratically, as the number of user pairs that could communicate,” Kovács said. “We found the critical number actually is a very small fraction compared to the number of users. But, if you add fewer than that, the network will fall apart, and people cannot communicate.”
Kovács sees this insight potentially aiding the development of a more robust and efficient quantum network. This network could adapt to failures by automatically replacing lost links with new ones, thereby enhancing its overall resilience.
“The classical internet was not built to be fully robust,” Kovács said. “It naturally emerged due to technological constraints and user behavior. It was not designed, it just happened. But now we can do better with the quantum internet. We can design it to ensure it reaches its full potential.”
Implications of Quantum Networks’ New Research and Future Applications
The implications of quantum entanglement for secure communications are profound. Quantum key distribution (QKD) allows for the creation of secure shared keys between parties, with any attempt at eavesdropping disturbing the quantum state and alerting the involved parties. This level of security is particularly valuable in industries such as finance and healthcare, where data sensitivity is paramount.
As quantum networks become more resilient, they could facilitate advancements in various fields. In healthcare, for example, the ability to transmit large volumes of data securely and swiftly could enhance telemedicine, enabling real-time consultations and data sharing among medical professionals. In finance, quantum networks could revolutionize risk assessment and fraud detection, allowing institutions to process vast datasets at unprecedented speeds.
However, the shift to quantum communication also presents challenges in cybersecurity, as quantum computing poses a threat to traditional encryption methods. This necessitates the development of quantum-resistant protocols to protect sensitive data.
The telecommunications sector stands to benefit significantly from quantum networks, which promise faster data transmission and ultra-secure communication systems. The prospect of a quantum internet, where classical and quantum communications coexist, could lead to a more efficient and secure networking environment.
Conclusion
The research conducted by Kovács and his team at Northwestern University represents a critical step toward addressing the challenges of stability and connectivity in quantum networks. Their innovative strategies could pave the way for a future where quantum communication is not merely a theoretical concept but a fundamental component of our digital infrastructure.
As we stand on the brink of a quantum revolution, the implications of these developments extend far beyond academia. The potential for a resilient quantum internet could transform industries, enhance security in communications, and fundamentally change how we interact with information. The journey toward realizing the full potential of quantum networks is just beginning, and with it comes the promise of a more interconnected and secure world.